The article below follows a most entertaining presentation to the Society on the evening of Thursday, 28th June 2018 from Professor Arthur Lowery, Director of the Monash Vision Group, as a joint lecture with the Victorian Division of the Australian Academy of Technology and Engineering (ATSE).
By Catriona Nguyen-RobertsonRSV Science Communications Officer
“Blindness is more feared by the public than any ailment with the exception of cancer and AIDS.” – William Dobelle, Artificial Vision Pioneer
Since the 1960s, people have been trying to stimulate the brain to restore vision. Many bionic vision devices involve replacing damaged photoreceptors with electrodes in the eye, however Professor Arthur Lowery bypasses the eye altogether and inputs signals for vision directly into the visual cortex of the brain to produce high-resolution images.Professor Lowery has been obsessed with batteries and valves since he was four years old. He broke many of his father’s drills, and built his first flashing light circuit at the age of seven (it still sits on his desk to this day). Today, he is an electrical and computer systems engineer leading the Monash Vision Group (MGV), an Australian Academy of Technology and Engineering (ATSE) fellow, and an Australian Research Council Laureate Fellow. His passion to strive in bionic vision science and technology may have stemmed from a young age, when he invented a cookbook with built-in lights for his grandmother who loved cooking but was hard of seeing.
Wonderful hearing about Prof Arthur Lowery @MonashUni & his wayward Yorkshire youth, breaking into the treasures in the disused "science cupboard" at high school with his matesl! Now an @atse_au fellow exploring the brain-machine interface & #bionic vision. pic.twitter.com/tcYiwVBrWz
— RSV (@RoyalSocietyVic) July 12, 2018
Recently, there has been worldwide “hype” around brain interfaces and the seemingly endless possibilities that they provide. Despite bold predictions from several technology companies about the future of neural interfaces (e.g. Elon Musk’s whole brain interface that will allow the brain to connect to an information network), the science of brain augmentation is still in its early days. There is a large gap between what is talked about amongst the hype and what is actually feasible in the near future. Professor Lowery gave us a reality check of what applications are possible for brain interfaces; electrodes can be placed to stimulate the occipital lobe for sight, the motor cortex for the control of prosthetics, or the speech centre for artificial speech. In addition, with bidirectional interfaces, it is possible to feed information into the sensory cortex to provide feedback as well as excitation signals. “Jump leads” can also be strung between different sections of the brain where connections may have been lost to bypass damaged areas (e.g. following stroke). There are multiple applications for this technology in the biomedical and engineering fields.Great progress in artificial vision has been made since the earliest works of Brindley and Dobelle in the 1960s and 1970s, however there remained significant clinical, neurophysiological, and engineering challenges to producing commercially available bionic vision devices. Dobelle developed a brain implant that films the visual field in front of the user with a digital camera and transmits a signal to 68 platinum electrodes on the surface of the visual cortex, similar to “a Band-Aid patch on the brain”. Not all of the electrodes worked, but it allowed patients to see outlines of figures. The greatest issue with this system was that a large amount of power was required to send signals inside the brain given that the electrodes were on the surface and far away from their target neurons, and using a greater amount of power resulted in occasional epileptic episodes. In 2016, Second Sight Medical implanted the first retinal prosthesis system, which uses a camera to capture images that are transmitted as a series of electrical pulses to an array of electrodes implanted around the eye.[caption id="attachment_4555" align="alignleft" width="300"]

Professor Arthur Lowery presenting to the Royal Society of Victoria[/caption]The Monash Vision Group (MGV) is a consortium of engineers and biomedical scientists, clinicians, and commercial companies led by Professor Lowery to develop bionic vision systems and build research capabilities in bionic vision science and technology. Since 2010, they have been developing the Gennaris bionic system, a wireless device designed to electrically stimulate neurons in the visual cortex. Tiles are placed around the visual cortex with electrodes penetrating 2mm into the surface, sending electrical input directly where the optic nerve would otherwise be sending signals. The benefit of penetrating electrodes is that, with a shorter travel distance, the electrodes require less power to transmit signals than the Dobelle model, also reducing the chance of causing epilepsy. Albeit, there have been other challenges in the development of the bionic eye that the MGV has had to overcome. Our brains were not designed with a big fibre-optic cable for terabytes of information to be transmitted directly into them. To optimise the device and determine the best possible methods of manipulating our neurophysiology, Professor Lowery has looked to “clever students, computers and experiments”.[caption id="attachment_4557" align="alignright" width="225"]
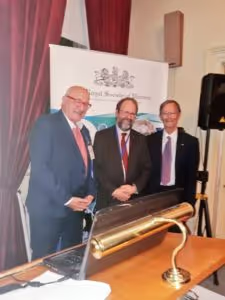
Professor Arthur Lowery (centre) with RSV President David Zerman (left) and ATSE Victoria Chair Dr Alexander Gosling AM.[/caption]It is difficult to place a single point of connection in the brain and receive a large amount of information. The state-of-the-art electrodes used in the Gennaris device are orders of magnitude larger than neurons, and the more electrodes are implanted, the weaker the structural integrity of the entire neuron network becomes. The electrodes themselves can also cause damage to surrounding neurons during stimulation. The Gennaris device therefore only has 43 electrodes with pointed ends per tile to be implanted on the visual cortex for maximum stimulation and minimum damage (up to 11 tiles may be implanted). Another consideration in the placement of the electrodes are the laws of physics: due to the spread of currents, the current density decreases as it spreads out from electrodes to target neurons, and therefore more current is required (which brings us back to the epilepsy problem). To overcome this, materials with large effective surface areas are ideal for efficient stimulation. Carbon nanotubes are an attractive candidate due to their outstanding electrical, chemical and mechanical properties, higher surface area, and high conductivity. Additionally, due to their smaller size, the dendrites of neurons (sensory extensions that receive input) readily branch out to form connections with the nanopillars directly.Gennaris comprises a miniature camera worn by the user on glasses, which can capture images and transmit them to the brain. The most useful features are extracted from high-resolution images using a number of processing techniques and converted to an electrical signal. The signal is transmitted to the implanted electrodes, which in turn, stimulate neurons in the primary visual cortex, evoking brief flashes of light, known as phosphenes, in the visual field of the user, which the brain interprets as images. A picture is thus formed from approximately 100,000 dots, or phosphenes, allowing for sufficient representation of the camera’s field of view – and can even detect the emotions of faces using emoticon software.After overcoming the hurdles of physiology, bioengineering, and “18th century physicists and their laws”, Professor Lowery and his team have generated a bionic vision system that has been approved for clinical trials. By implanting electrodes directly within the visual cortex, the device bypasses damage to the eye and optic nerve, therefore addressing a large percentage of causes of incurable blindness with relatively high resolution.
Discover how you can join the society
Join The Royal Society of Victoria. From expert panels to unique events, we're your go-to for scientific engagement. Let's create something amazing.
